The ability to see red as a separate color has evolved independently at least 4 times in butterflies.
Background
Vision is based on the perception of light, a process that begins when light enters the eye of an organism such as an insect or a human. Cells inside the eye called photoreceptors detect that light using a specialized protein called an opsin. Each opsin is sensitive to its own set of wavelengths, and we can plot a graph of its sensitivity as a function of wavelength, producing a shape that resembles a bell curve. The peak of this curve, the wavelength that the opsin is most sensitive to, is its peak wavelength, also denoted λmax or lmax.
Wavelengths of visible light are most often measured in nanometers or nm for short. One billion nm equals one meter. Visible light extends conservatively between 400nm violet and 700nm red, although it is possible to see bright light a little bit beyond this range. I will be giving opsin λmax values in nanometers as well. The colors we see for different wavelengths are roughly: violet < 440nm; indigo 440-460nm; blue 460-480nm; blue-green 480-520nm; green 520-550nm; yellow-green 550-570nm; yellow 570-590nm; orange 590-610nm; red > 610nm.
Familiar Colors
Human vision uses 4 kinds of photoreceptor having 4 kinds of opsin. Three of these are the cone cells, functioning in bright light and affording not only color vision but also high resolution vision. The fourth type consists of the rod cells, most sensitive to blue-green light and mainly used for seeing in darkness. Here is a graph of the human photoreceptors' responses to wavelength:
The most common type of color vision is believed to adhere to the opponent process theory. The gist of this is that the neurons of the eye and/or brain compare signals from different photoreceptors with different wavelength sensitivities, producing color perception by categorizing each color as favoring one or the other receptor. In humans, the opponencies are red-green and yellow-blue; every color falls somewhere along both the reddish-greenish continuum and the yellowish-bluish continuum simultaneously, and no color can ever be reddish-green or yellowish-blue. The opponent processes can also be plotted by wavelength:
The red-green opponency is a comparison between the two kinds of cone we call "red" and "green", while the yellow-blue opponency compares blue-sensitive cones and the sum of the signal from the other two kinds. Notice that brightness also acts like an opponency, and it depends only on red and green cones in bright light and rods in dim light. The reason blue cones don't contribute to brightness is there aren't enough of them, and they're too big and far between. By limiting brightness to the smaller, tightly packed red and green cones, we have better visual acuity (resolution) than we would otherwise.
In the middle of each color opponency is a neutral wavelength, where the perceived color is neither reddish nor greenish (neutral yellow) or where the color is neither yellowish nor bluish (neutral green). It is possible to identify neutral yellow and neutral green in humans, by looking at single wavelengths of light and then making the light progressively brighter.
Making red light brighter causes it to look more orange, then yellow, then it becomes painful to look at before seeming to turn white. This is because the "red" cones reach an upper limit of how excited they can be, while "green" and ultimately "blue" cones become more and more excited. Violet light also seems to turn more and more blue as brightness is increased, due to "blue" cones reaching their limit while "green" cones keep responding more. But at extremely short wavelengths the violet light only seems to become grayish, probably due "red" and "green" cones having nearly equal sensitivities at such wavelengths.
But certain wavelengths don't appear to change color, instead only seeming to turn white. These are the invariant hues: 574nm yellow, 507nm green, and 476nm blue. That means what we normally think of as yellow (about 580nm) is really slightly orange, and the hues we think of as green are mostly yellow-greens. Primary red doesn't occur anywhere on the spectrum, because every wavelength we call red is at least somewhat orangeish. The fact that violet becomes blue as brightness increases, and the existence of an invariant violet somewhere around 400nm, indicate that violet, not blue, is the third primary color, and what we call blue is really greenish violet.
Humans are usually trichromatic, meaning we see in three primary colors. But for this document I want to reframe chromacy in a different way. I want to characterize it by how many opponent processes an organism has. Humans usually have two color opponencies plus one for brightness, that makes three, therefore humans are usually trichromatic. An animal with only one color opponency, such as a cat or dog, would be dichromatic (di meaning two), and one with three color opponencies, such as most birds, would be tetrachromatic (four primary colors). The reason I want to describe chromacy this way is because some animals don't have the direct one-to-one relationship between the number of photoreceptors and the number of primary colors that humans do.
I will be using terms for higher numbers of chromacy, such as pentachromacy (5 primary colors), hexachromacy (6 primary colors), and heptachromacy (7 primary colors). This number is sometimes less than the number of photoreceptor types.
Insect Color Vision
Most insects are trichromatic, like humans. But instead of red, green, and blue, their primary colors are green (or yellow), blue, and ultraviolet. Inside the insect eye the photoreceptor cells are clustered together with several cells forming an outer ring and one or two cells in the middle. The outer ring is usually made up of green-sensitive cells. For this reason I will call it the green ring. It seems to not only participate in color vision but also forms the main sensor for brightness and shape, and is most likely the only set of receptors active in dim light. So the green ring receptors not only fill the same role as our red and green cones, but probably also the same role as our rods.
Importantly, each hexagon of an insect's compound eye does not form its own image. So those "bug eye" toys from the 1980s and 1990s were just plain wrong about how insects see. Each hexagon, called an ommatidium, funnels the light waves coming from one direction and directs them to the photoreceptors in the back. In technical language it acts like a waveguide, preventing an image from forming by making all the light move in the same direction. In fact, the green ring is probably all wired together onto one neuron anyway, since that offers the best sensitivity in low light, so even if there were a tiny little image there the bug still wouldn't see it. So each ommatidium represents a "pixel" of insect vision, one individual dot out of the whole of what it sees. Insect vision, then, is not very detailed at all.
Behavioral studies on honeybees have shown that they perceive color using opponent processes, just like we do. These studies showed that bees see yellow and blue as opponents, consistent with having an opponency between the green-sensitive and blue-sensitive cells, and blue-green as opponent to ultraviolet, consistent with having an opponency between the green and ultraviolet photoreceptors. So we can deduce that in insect vision, each of the cells in the center is opponent to the green ring. Genetically, the blue-sensitive opsin evolved from the UV opsin, and insects evolved from crustaceans*, and most crustaceans have a green ring with only a single UV cell in the middle, so the evolution of seeing blue must have come with a duplication of the green-UV opponent system to form a green-blue system.
*Scientists are even saying nowadays that insects are a type of crustacean.
Those studies also showed that bees ignore brightness, meaning they either don't see it or don't care. Other insects do respond to brightness, although it might depend on the species whether they use the green ring or the UV photoreceptor to sense it. Fruit flies presented with two tunnels, one leading to a green light and one to a UV lamp, always take the UV path, but flies engineered to lack the UV receptor choose the green light instead. But maybe fruit flies simply like the color UV.
In the case of butterfly vision, when red receptors are present they can be expressed in a few different patterns. One is that some of the cells of the green ring are red-sensitive cells instead, with separate neural connections for the red and green signals. Another is that the green rings of some ommatidia may contain ony green cells, others only red cells, and maybe still others a mixture of both. Or the red receptor could be inside the middle of the ring along with the blue and UV receptors.
But another way is through tiering, which is where the cells are stacked up together. Normally a photoreceptor cell is a long thin object adapted to catch as much light as possible from as small an area as possible. But if two long thin cells are placed end to end, light will reach one of them first before passing into the other. Opsins absorb light, it's how they do what they do, so the light that goes into the second cell has a little bit different spectrum than what goes in the first cell, and the two cells will be sensitive to slightly different wavelengths. The second cell might have a different opsin sensitive to longer wavelengths, and/or there may be a light filter, called a screening pigment, between them.
For this document I have opted to treat red and green as opponents for all insects that have red sensitive cells. To predict the neutral points I've used my Animal Vision Interactive Analyzer utility, a.k.a. AVIAN. I will give neutral yellow wavelengths for the different kinds of butterflies in order to compare them. The shorter the wavelength of neutral yellow, the more things like green plants will look yellow, brown, or orange, the less vivid red objects will appear, and the more washed out green objects will look. Humans are moderately good at distinguishing red and green with our 574nm neutral yellow, surpassing nearly all butterflies, but pigeons have their yellow all the way out at 600nm! Most birds are much better at color vision than we are.
The Simplest Butterfly Red Vision
The gossamer winged butterflies (Lycaenidae), and their close relatives the metalmark butterflies (Riodinidae), have an extra opsin sensitive that lets them see red light. Depending on the species, this opsin has a λmax anywhere between 568nm and 600nm. However, their neutral yellow is predicted to be between 535-565nm, depending on the species, which is an awfully short neutral wavelength to be calling it a red-green opponency. This means that some things that look green to humans might look yellow or even orange to these butterflies, such as green plants.
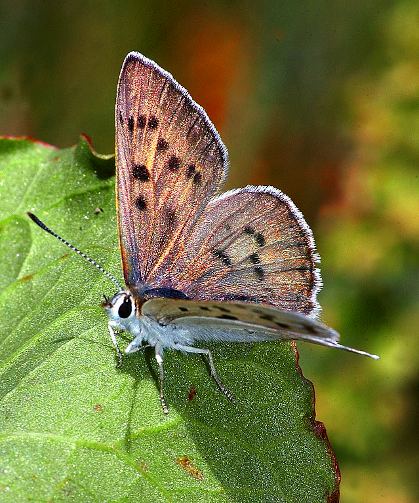
Photoreceptors and predicted opponencies of the blue copper (Lycaena heteronia).
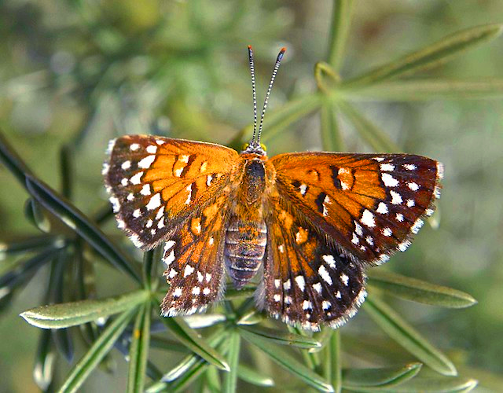
Photoreceptors and predicted opponencies of the Mormon metalmark (Apodemia mormo).
The holly blue (Celastrina argiolus) seems to have lost its blue-green opsin, reverting back to the kind of trichromacy most insects have. Its "red" receptor has even shifted down to 560nm, as if to fill the gap in the rainbow.
Filtered Light
The brush footed butterflies (Nymphalidae) do not have a fourth opsin, but many species use a screening pigment to filter out all but red light. By expressing the same green-sensitive opsin in different cells with or without the filter, they get a fourth type of photoreceptor and can distinguish red and green light. Species that don't have a screening pigment have their green-sensitive opsin λmax around 530nm, similar to the human green cone and not very effective for seeing red light, while those that do have the filter have that opsin shifted to a longer wavelength to take advantage of the filter.
The monarch butterfly has a green opsin λmax at 545nm. The combination of this opsin with the screening pigment makes a red receptor most sensitive to about 600nm. Keep in mind that the filter doesn't make the cell more sensitive to red light, it only limits the wavelengths it has access to. It's still the same opsin curve in there, but for this graph we cranked up the intensity so the curves would all be equal height. The filter actually blocks most of the light the opsin could respond to, meaning these butterflies can only see red objects when it's plenty bright out, such as on a sunny day. Around dawn and dusk their eyes would have a hard time seeing red, making red objects look very dark, and the whole world would probably take on a greenish hue.
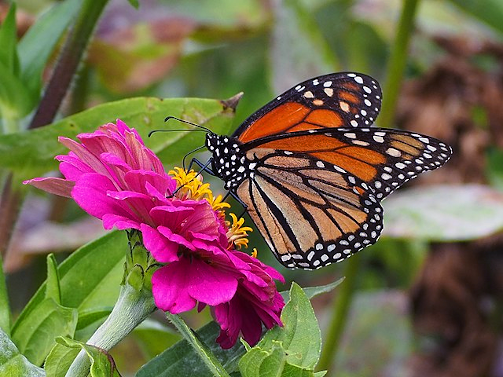
Photoreceptors and predicted opponencies of the monarch butterfly (Danaus plexippus).
The filtering in the graphs for the monarch butterfly are educated guesses. A monarch screening pigment hasn't been directly measured, but behavioral evidence shows they can distinguish colors that require a red receptor. Some species in this family posess a red receptor and others apparently don't, but the ones that do are spread throughout the evolutionary tree, meaning the common ancestor probably had a red receptor and some of the species may have lost it for whatever reason.
Extra Red
Certain butterflies of the Pieiridae family have a more advanced color vision system. The cabbage white and garden white have the capacity to tell apart two different shades of red - as separate primary colors! This is done by filtering a single 560-565nm opsin, using it to make three different kinds of photoreceptor: one sensitive to green, one to orange-red (600-620nm depending on species), and one to purer red (about 635nm). What are they looking at that they benefit from having two different kinds of red receptor? Besides this amazing development, they also have a violet receptor with its own violet-sensitive opsin. This makes them capable of hexachromacy.
The neutral yellow for the cabbage white is predicted to be at 577nm, very similar to the human 574nm yellow. But they are predicted to also have an additional red-green process with a neutral wavelength of about 610nm. That means any given wavelength in between the two, like say the light from a sodium vapor lamp or an amber LED road construction light, would look like some kind of bizarre half-green version of yellow-orange. Like the brush-footed butterflies, pieirid red receptors wouldn't work very well in dim light, causing the same greenish cast and apparent darkening of red objects.
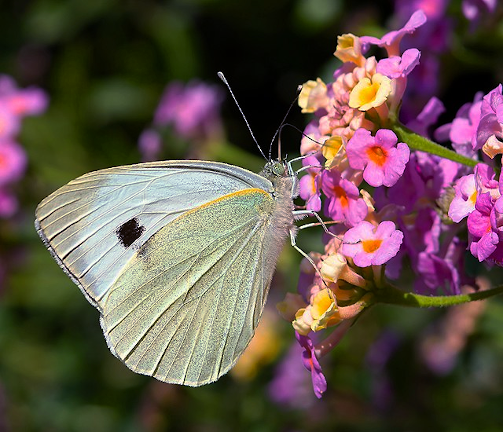
Photoreceptors and predicted opponencies of the cabbage white (Pieiris brassicae).
Extreme Color Vision
The swallowtail butterflies (Papilionidae) have the most advanced color vision known of any insect. Most have a system of four opsins and two screening pigments, combined to make 8 kinds of photoreceptor. Three of these contain multiple opsins, making them good broadband receptors. One receptor is dedicated to sensing red light, using either a 560nm opsin or a 575nm opsin, subtly filtered, and if it forms an opponency with the green receptor then the neutral yellow ranges from 540nm-565nm across multiple species. The reduced red sensitivity at dusk and dawn would not affect these butterflies nearly as much as the cabbage white or the monarch. Other receptors are sensitive to blue, violet, or ultraviolet, but since the violet receptor uses the UV opsin with a filter, it would be less sensitive in dim light, whatever effect that may have on their color perception.
The Japanese swallowtail is one of the more extensively studied swallowtail butterflies and its predicted color opponencies make it at least tetrachromatic, and probably weakly hexachromatic. Its 550nm neutral yellow means the world would look quite a bit more orange/beige than it does to humans, with green plants appearing yellow.
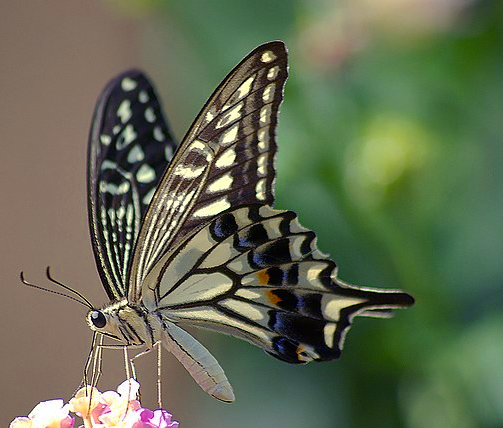
Photoreceptors and predicted opponencies of the Japanese swallowtail (Papilio xuthus).
But one species of swallowtail, the golden birdwing, actually has even more sophisticated color vision than the others. It has no less than nine kinds of photoreceptor, containing six different opsins, four of which are sensitive to green/yellow/red light. It is probably pentachromatic, and maybe weakly heptachromatic (seven primary colors). This makes it quite a specialist for red-green vision, and its neutral yellow is predicted to be 586nm.
Photoreceptors of the
golden birdwing (Troides aeacus formosanus).